Astronomers have long been captivated with neutron stars, which are dense remains of supernova explosions, because of their extraordinary characteristics, especially their strong magnetic fields. These stellar remnants are not only fascinating due to their density but also due to the incredible physical processes that occur within them. Neutron stars are so dense that a sugar-cube-sized amount of their material would weigh about as much as all of humanity. The creation of these magnetic fields has been clarified by recent study conducted by Dr. Andrei Igoshev of Newcastle University, particularly in low-field magnetars, which are neutron stars with comparatively weaker magnetism. The complexities surrounding neutron stars and their magnetic properties continue to inspire ongoing research, leading to discoveries that challenge our understanding of physics.
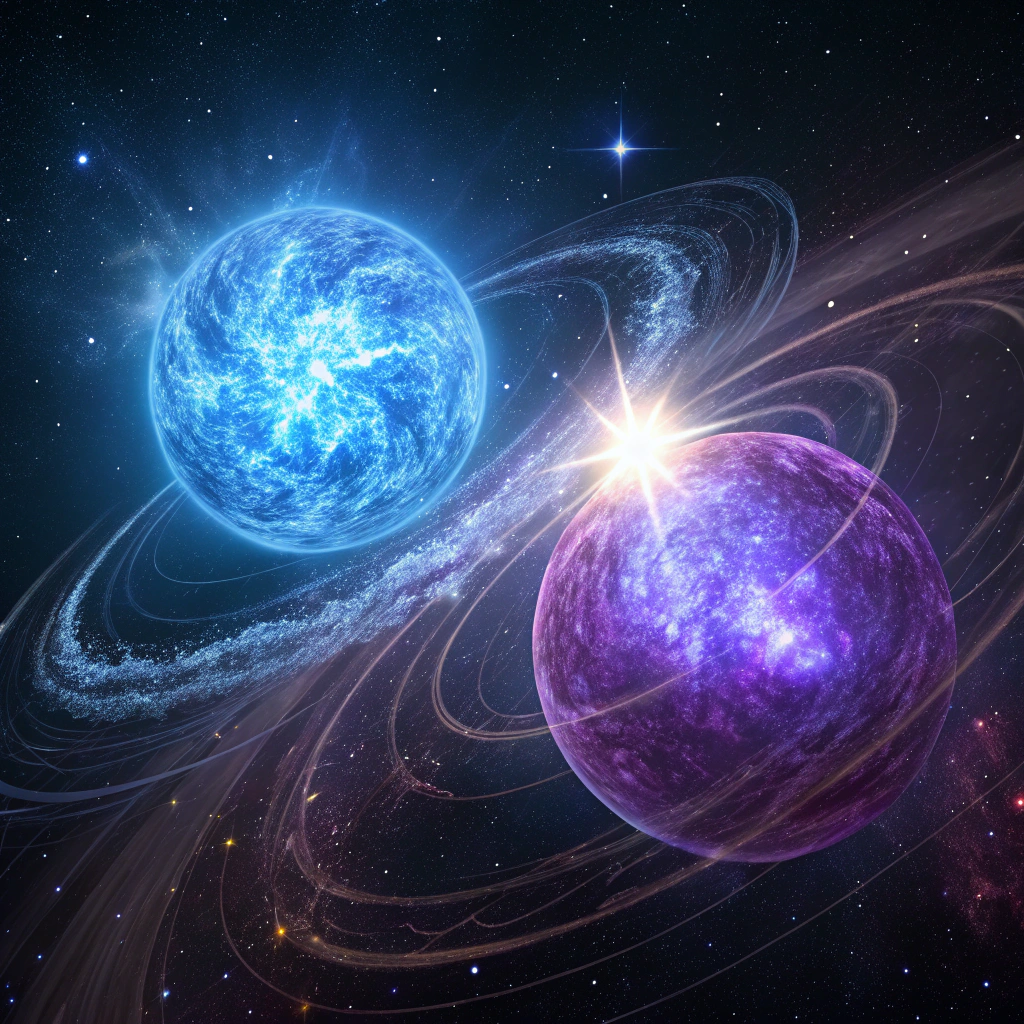
The majority of a big star’s outer layers are ejected into space during a supernova explosion, marking the birth of a neutron star. This explosive event is one of the universe’s most violent phenomena, resulting in a spectacular display of light and energy. The freshly generated neutron star may spin faster if part of the material that was expelled falls back upon it, a phenomenon known as fallback accretion. Through a process known as the Tayler-Spruit dynamo mechanism, this rapid rotation is essential to the creation of magnetic fields. The Tayler-Spruit dynamo describes how the motion of the star and its internal fluid dynamics can generate substantial magnetic fields. Only lately have computer models been used to replicate this technique, which was first postulated about 25 years ago. These models allow scientists to simulate various scenarios and understand the conditions necessary for magnetic field formation. With an internal strength significantly higher than the exterior one, the resulting magnetic field is complicated and intricate, revealing much about the star’s internal dynamics.
The enormous magnetic fields of magnetars, a kind of neutron star, are hundreds of billions of times greater than the magnetic field of Earth. This immense strength enables magnetars to influence their surroundings significantly, affecting nearby matter and even emitting bursts of high-energy radiation. Magnetars are brilliant, fluctuating sources of X-ray radiation because of these strong fields, making them some of the brightest objects in the universe. It’s interesting to note that some neutron stars with lesser magnetic fields are categorized as low-field magnetars because of their similar X-ray emissions. The creation of these weaker magnetic fields can be explained by the Tayler-Spruit dynamo process. Researchers are continuously studying the relationship between these magnetic fields and the various forms of radiation emitted by neutron stars, as this could provide insights into fundamental astrophysical processes.
To better study the intricate magnetic fields of neutron stars, Dr. Igoshev is starting a new research team at Newcastle University, bringing together experts in astrophysics, computational modeling, and observational astronomy. This interdisciplinary approach aims to tackle the complexities of neutron star physics by combining theoretical models with observational data from telescopes around the world. Knowing these magnetic fields helps us better understand the physics underlying some of the universe’s most extreme conditions while also advancing our understanding of neutron star formation and evolution. The research not only contributes to our knowledge of neutron stars but also has implications for broader astrophysical phenomena, including the formation of galaxies and the lifecycle of stars.
This study provides a better understanding of how some neutron stars develop their magnetic characteristics, which is a major advancement in astrophysics. Understanding these processes is crucial, as it could reveal connections between neutron stars and other cosmic entities, such as black holes and white dwarfs. We might expect ever more in-depth understandings of the mysterious nature of these celestial objects as computer models advance. As technology progresses, advancements in observational techniques and computational power will allow scientists to explore neutron stars in unprecedented detail, leading to new discoveries and insights into the universe’s workings.
In conclusion, neutron stars represent one of the most intriguing areas of research in modern astrophysics. They challenge our understanding of physics and provoke questions about the fundamental nature of matter and energy. By studying neutron stars and their magnetic fields, researchers not only seek to unveil the mysteries of these celestial objects but also to understand the broader implications for the universe. The ongoing research led by Dr. Igoshev and his team at Newcastle University is a promising step towards unraveling these enigmas, paving the way for future discoveries that could reshape our understanding of the cosmos.
Moreover, public interest in neutron stars continues to grow, spurred by advancements in technology and increasing accessibility to astronomical discoveries. As images and data from powerful telescopes like the Hubble Space Telescope and the upcoming James Webb Space Telescope become available, the general public can engage with scientific research in ways never before possible. Educational initiatives and outreach programs aimed at informing the public about neutron stars and their significance in astrophysics can inspire the next generation of scientists and astronomers, ensuring that the quest for knowledge about the universe continues.
Additionally, the study of neutron stars could provide insights into fundamental physics, particularly in the field of quantum mechanics and general relativity. For example, the extreme conditions present in neutron stars may give rise to phenomena that cannot be replicated in laboratory settings on Earth, offering a natural laboratory for scientists to test theories of physics under extreme conditions. Furthermore, understanding the life cycle of neutron stars and their eventual fate as they collide or merge with other stars can shed light on the origins of heavy elements in the universe. Such research not only expands our knowledge of these dense objects but also contributes to our understanding of the universe’s evolution as a whole.